Preterm Birth Effects on Visuospatial Working Memory
Neural alterations of the Visuospatial Working Memory Network in Children Born Preterm: A Systematic Review
Abstract
Today premature birth has been identified as a serious clinical and public health issue that modern medical knowledge has failed to tackle. Quite the contrary, rates of preterm birth are rising globally. It is well-established that children born preterm exhibit deficits in most cognitive domains, most prominently working memory with a specific vulnerability in visuospatial processing. Although there is substantial evidence on the neural basis of visuospatial working memory (VSWM) in typically developing individuals, little is still understood with respect to this matter in at-risk populations, such as those born preterm, during brain development. Yet working memory skills have been found to underlie higher-order cognitive abilities, such as executive function, while playing a mediating role in later academic attainment and social competence. The goal of this review was to provide an overview of the existing literature regarding the neural correlates of the visuospatial working memory system of preterm children, from infancy to adolescence, over the last decade. Both structural and functional changes are reported in most studies, while the recruitment of compensatory mechanisms results in a catch-up effect at a behavioural level. With this information, early targeted interventions can be designed in order to optimize outcome in this population.
Introduction
Preterm children (PT) are those of a gestational age of less than 37 weeks. A recent publication of the World Health Organization reported that approximately 15 million preterm infants are born every year around the world (World Health Organization, 2016). Over the last few years, there has been a dramatic increase in the rates of survival of preterm children, yet premature birth still is the primary cause of infant mortality and morbidity in developed countries (Goldenberg, Culhane, Iams & Romero, 2008). Even though, only a small percentage of preterm children present with serious disabilities, such as cerebral palsy and sensory impairment, there is a larger group suffering from subtler deficits in domains including executive function, behavioural and emotional problems and learning disabilities (for review see Anderson, 2014). These difficulties can largely affect the child’s academic attainment and general well-being and may persist into adulthood (Taylor, Minich, Bangert, Filipek & Hack, 2004). Therefore, an in-depth understanding of the long-term sequelae following premature birth is needed in order for this high-priority public health issue to be addressed and effectively managed.
Effects of prematurity on early brain development
Premature birth occurs in a period critical for the normal maturation of the central nervous system. In fact, preterm birth is known to have a “double jeopardy” effect on early brain development, since it not only interrupts the typical process of brain development in utero, but also exposes the infant to pathological conditions, such as periventricular leukomalacia, which would not normally be encountered at this gestational age (Schonkoff, Phillips & National Research Council, 2000). Such conditions cause early brain lesions usually affecting subcortical areas, such as the thalamus, hippocampus, caudate nucleus, the frontal cortex and the corpus callosum, while disrupting myelination processes, leading to both white and grey matter damage (Luciana, 2003). Recent advances in neuroimaging have allowed researchers to study altered brain structures and function of the preterm brain in more detail. A meta-analysis by de Kieviet, Zoetebier, Van Elburg, Vermeulen and Oosterlaan (2012) suggests that the preterm brain has significantly smaller total, white and grey matter volumes. These structural differences have been linked to poorer IQ, working memory, language and executive function by other researchers (Allin et al., 2004; Taylor et al., 2011). However, compensatory factors, such as brain plasticity and social factors play an important role in determining final outcome (Sansavini et al., 2007).
Working Memory and Preterm Birth
A large body of evidence shows that preterm-born children are at higher risk for developing mild cognitive deficits related to memory and executive functions (de Haan, Bauer, Georgieff & Nelson, 2000; Soria-Pastor et al., 2008), academic underachievement and behavioural problems (Aarnoudse-Moens, Weisglas-Kuperus, van Goudoever & Oosterlaan, 2009; Anderson, Doyle & Victorian Infant Collaborative Study Group, 2003). Working memory (WM), in particular, has been identified as a strong predictor of academic achievement in children born preterm (Gathercole, Alloway, Willis & Adams, 2006; Mulder, Pitchford & Marlow, 2010). In the classic definition by Baddeley and Hitch (1974), working memory is defined as the ability to mentally store and process information over a brief period of time. Up to date, the most influential theoretical account of WM is the three-component model described by the same authors(Fig 1). The neuroanatomical dissociation between phonological and visuospatial working memory is supported by multiple findings of imaging studies (Gruber & von Cramon, 2003; Haxby, Petit, Ungerleider & Courtney, 2000). Additionally, Hamilton, Coates and Heffernan (2003) proposed a dual-stream subserving the visual and spatial components of the visuospatial sketchpad, the former being in place earlier than the latter.
Figure 1
: Baddeley’s three-component model of working memory .
Nevertheless, deficits in VSWM are a convergent finding in the preterm literature and might explain the problems that preterm children encounter in terms of behaviour and learning (Fitzpatrick, Carter & Quigley, 2016; Luciana, Lindeke, Georgieff, Mills, & Nelson, 1999). In fact, Gathercole and Alloway (2008) reported that typically developing children with working memory deficits also face problems in learning domains, such as reading, spelling and mathematics. Lastly, WM deficits have been repeatedly associated with a number of developmental disorders, including autism and attention deficit disorder (Barendse et al., 2013; Martinussen, Hayden, Hogg-Johnson & Tannock, 2005). However, the nature of these impairments is still poorly understood, which has previously been attributed to varied methodologies and definitions employed by different studies (Lee, 2015). It is thus easily understood why knowing the neural substrate of VSWM in preterm children comes with considerable advantages. Not only will it inform research by contributing to the increasing understanding of the relationship between neonatal brain lesions and later neurocognitive outcome, but also guide the design and delivery of early and targeted intervention plans by clinicians in order to use children’s strengths to facilitate potential areas of weakness and optimize outcomes. Thus, aim of this systematic review was to examine the existing literature and shed light on the neural correlates of VSWM impairment observed in several studies at a behavioural level. The reason why this paper focused specifically on visuospatial and not verbal working memory is the selective nature of these deficits with verbal WM usually being spared (Rose, Feldman & Jankowski, 2011).
METHOD
Identification and study selection
A literature search was conducted in two major databases for Psychology Articles, PubMed and PsychINFO, using the search terms “preterm”, “prematur*”, “low birth weight” and “working memory” or “executive function”. The results of these searches were 65 articles from PubMed and 73 from PsychINFO. Further articles were handsearched in these databases. After eliminating duplicates, 45 articles remained and were further studied according to the following inclusion and exclusion criteria(Fig 2):
- Studies published between June 2005 and June 2017 in English-language peer-reviewed journals.
- Articles employing neuroimaging methods or using imaging data collected at different time points.
- The use of VSWM measures in childhood and adolescence (up to 18 years) and reported sample sizes, test score means and standard deviations for each group. Measures that tapped on phonological working memory or short-term memory exclusively were not included.
- Studies of sufficient quality determined by the STROBE checklist (Vandenbroucke et al., 2007). This tool comprises of 22 items assessing among other, methodological quality, external validity and presentation of results (See Appendix).
- Case studies, outcome studies about working memory training or other therapeutic interventions and publications on methodology and/or validation of executive functioning tests were excluded.
- Studies that included different age groups were included only if they reported results in childhood and adolescence separately from adulthood.
- Studies that examined executive function outcomes were considered only if separate analyses were conducted for VSWM scores.
Figure 2
: flow-chart of the included and excluded studies.
Methodological Quality
All the studies included in the systematic review were fully read and assessed according to the STROBE checklist for cross-sectional, cohort and case-control studies (see Appendix). Most studies were of sufficient to high methodological quality, only two did not include an age- and sex-matched control group (Beauchamp et al., 2008; Scratch et al., 2015) and two more had a relatively small sample, which could affect generalizability and robustness of findings (Curtis et al., 2006; Taylor et al., 2012). All studies that employed magnetic resonance imaging (MRI) at term-equivalent age were longitudinal, four studies were cross-sectional and used functional magnetic resonance imaging (Curtis et al., 2006; Griffiths et al., 2013; Mürner-Lavanchy et al., 2014; Taylor et al., 2012). All fMRI studies administered VSWM tasks during scanning, but only Curtis et al. (2006) and Mürner-Lavanchy et al. (2014) administered additional assessments outside the scan. Lastly, all but two studies (Taylor et al., 2012; Thompson et al., 2013) took confounding socioeconomic and medical-perinatal factors into account when interpreting findings.
Classification of preterm children
Preterm-born children were considered those of a gestational age (GA) of less than 37 weeks and low birth weight of less than 2500g. Preterms were further divided into 2 categories according to age of gestation: late or moderate preterm (LPT) with a GA between 34-36 weeks, very preterm (VPT) between 33-29 weeks and extremely preterm (EPT) with a GA of 28 weeks or less (Engle, 2004).
Table 1: Studies examining the neural correlates of visuospatial working memory in children born preterm. |
|||||||||
Reference |
Design |
Sample Size |
PT Age in yrs (M, SDs) |
PT BW in gr (M, SDs) |
Working Memory Measure |
Neuroimaging Technique |
Main Results |
SES factors controlled |
|
PT |
FT |
||||||||
Woodward et al., 2005 | Longitudinal | 92(<32w) | 103 | 2(0.16) | 1088 (315.2) | MLMS task | MRI (TEA) | PT infants showed greater difficulty in encoding new information in their working memory than controls. This finding was associated with reduced global and regional brain tissue (especially in dlPFC, sensorimotor, parieto-occipital and premotor cortex) at term equivalent age. | Maternal age and education, family income. |
Curtis et al, 2006 | Cross-sectional | 9(<32w) | 9 | 13.8(1.3) | 1,519.2 (402.1) | Modified CANTAB: spatial span task | fMRI | PT performance similar to FT. However, during the encoding phase of the spatial memory span task, the PT sample exhibited higher activation in the right caudate nucleus and less right activation during the test phase. No association between smaller hippocampi and memory outcome were found may be due to the effect of small sample size. | Parental education, average household income |
Beauchamp et al., 2008 | Longitudinal | 227(<32w) | N/A | 2 | 957 (218) | Delayed alternation task | MRI (TEA) | VPT children had significantly smaller hippocampal volumes shortly after birth, which has a lasting effect on visual working memory. | Family structure, parental education, occupation and employment status. |
Clark & Woodward, 2010 | Longitudinal | 103(<32w) | 108 | 6 | 1,066 (316.27) | Corsi Blocks | MRI (TEA) | VPT children with mild to moderate-severe cerebral abnormalities were particularly vulnerable to VSWM impairment regardless of social or clinical background factors. | Family structure, maternal age at birth, household income |
Taylor et al., 2012 | Cross-sectional | 10 (<32w) | 10 | 7-9 | N/A | n-back task | fMRI | No behavioural performance difference between the test and control group. However, the PT group showed no significant activation in the frontal regions, right hippocampus and left precuneus during this task. | N/A |
Griffiths et al., 2013 | Cross-sectional | 28(<28w) | 28 | 11 | 898 (154) | Combined n-back with Stroop task | fMRI | Performance on a working memory/attention task was lower for the PT group and associated with brain activation on different brain areas (prefrontal, parietal areas and the anterior cingulum) compared to FT. | Maternal education |
Thompson et al., 2013 | Longitudinal | 227 (<30w) | 46 | 7.5 (0.2) | 964 (219) | CMS: Dot Locations | MRI (TEA) | VSWM deficits in VPT, and various hippocampal shape differences. No correlations between hippocampal shape and memory outcome. Left hippocampal volume at term correlated with visual memory outcome, but not after correcting for intracranial volume. | N/A |
Mürner-Lavanchy et al., 2014 | Cross-sectional | 41(32w) | 36 | 9.83 (1.61) | 1286.92 (356.54) | MLT-C: Visuospatial Shape Location, Dot Location Task | fMRI | PT exhibited similar WM performance with CG but less frontal activation, especially in the ACC. Low-performing and younger PTs employed an atypical VSWM network compared to older and high-performing PT, suggesting that typical VSWM network is established by 12 years. | Parental education |
Omizzolo et al., 2014 | Longitudinal | 198 (<32w) | 70 | 7.5 (0.02) | 960 (222) | WMTB-C: Block Recall, CMS: Dot locations | MRI (TEA) | Working memory deficits found at a behavioural level. Increasing severity of neonatal global brain abnormalities, especially in deep grey matter (thalamus and basal ganglia), is linked to poorer VSWM performance and learning at age 7. | Family structure, language spoken at home, maternal age at birth, parental education, occupation and employment status. |
Scratch et al., 2015 | Longitudinal | 83(<32w) | N/A | 7.5 (0.7) | 975 (235) | WMTB-C: Block Recall, CMS: Dot Locations | MRI (TEA) | Higher GH levels during the first 6 postmenstrual weeks were linked to poorer VSWM and spatial learning at age 7 years as well as larger amygdala volumes after controlling for potential confounders. Visuospatial memory skills particularly vulnerable to a period of aberrant GH levels | Family structure,maternal age at birth, language spoken at home, parental education, occupation and employment status. |
Vollmer et al., 2017 | Longitudinal | 134(<36w) | 94 | 18 (0.17) | 1049 (256) | Corsi blocks (WAIS-III NI) | MRI(15.2) | In the PT group no correlation was found between GM or WM volumes and visuospatial working memory. However, there were significant correlations between working memory scores and microstructure of multiple WM tracts, which were not observed in the FT group. | Residential area, schooling, parental education and mother’s age at birth |
Test abbreviations
:
CANTAB= Cambridge Neuropsychological Test Automated Battery, CMS= Children’s Memory Scale, MLMS= Multilocation Multistep, MLT-C= Memory and Learning Test battery for Children, WAIS-III NI=Wechsler Adult Intelligence Scale 3rd edition as a Neuropsychological Instrument, WMTB-C= Working Memory Test Battery for Children
Other abbreviations:
PT=Preterm, T= Term, BW= Birth Weight, M=mean, SD= Standard Deviation, N/A= Not Appicable, MRI= Magnetic Resonance Imaging, fMRI= functional Magnetic Resonance Imaging, TEA= Term-equivalent Age, CG = Control Group, WM = Working Memory, VSWM= Visuospatial Working Memory, dlPFC= Dorsolateral Prefrontal Cortex, ACC= Anterior Cingulate Cortex, GH= Growth Hormone, SES= Socioeconomic Factors,
RESULTS
The literature search yielded 11 articles that were included in the present review (Table 1). Despite converging evidence of early brain abnormalities in PT children, only few studies have investigated the association between neonatal brain damage and long-term cognitive outcome. Amongst them, Omizzolo and colleagues (2014) examined the relationship between neonatal cerebral pathology and later memory and learning function at school-age. Magnetic resonance imaging (MRI) was obtained at term-equivalent age and a large cohort of VPT children (N=198) was subsequently assessed at the age of 7. Findings revealed that VPT children exhibited difficulties in all memory subdomains, including VSWM, even after controlling for social risk and below-average IQ. Specifically, the authors suggest that VPT children are three times more likely to exhibit WM impairment, while severity of global brain abnormality, especially in deep grey matter(thalamus and basal ganglia) adversely affects performance and learning. Complimentary findings come from two studies; one by Woodward et al. (2005), reporting that both global and regional brain tissue reductions (dlPFC, parieto-occipital cortex, premotor and sensorimotor cortices) and additionally quality of white matter connectivity at term were the best predictors of VPT infants’ performance at 2, and the second by Clark and Woodward (2010), who confirmed the association between cerebral abnormalities of varied severity and VSWM impairment, regardless of medical or social background. Beauchamp et al. (2008) examined the same question in preterm infants, using a more visually-dependent delayed alteration task. Contrary to expectation, no correlation between dorsolateral prefrontal, parietal or occipital volumes and task performance was found. In contrast, a strong link between hippocampal volume reduction and poorer visual WM was revealed, suggesting that VSWM in preterm infants is strongly reliant on the structural integrity of the hippocampus. Thompson et al. (2013), also, focused on the hippocampal region using MRI in the same age group. They found structural differences suggesting white matter injury at term, but failed to predict WM measures 7 years later. One possible explanation for these findings was provided recently from an original study by Scratch et al. (2015), who gathered postnatal physiological data in a cohort of VPT infants and reached the novel conclusion that higher concentrations of growth hormone, commonly found in preterm infants over the first days of life, were associated with VSWM outcome at school age and structural changes in subcortical areas. Indeed, growth hormone therapy given to PT children born too small for their GA has been previously related to overall cognitive and behavioural improvement (van Pareren, Duivenvoorden, Slijper, Koot & Hokken-Koelega, 2004). The most recent study included in the review is the one by Vollmer et al. (2017), who used fractional anisotropy (FA) to investigate associations between alterations in white matter microstructure and executive in preterm born adolescents in the absence of perinatal brain damage. Group comparison yielded underperformance of the preterm group in measures of VSWM, significant differences in white and gray matter volumes and diffuse alterations in FA. Subsequent analysis revealed no association between structural differences and behavioural performance, whereas significant positive correlations were found between WM and most of the affected white matter tracts of the brain exclusively in the preterm group, including the uncinate fasciculus, part of the internal capsule and both the inferior and superior longitudinal fasciculus. Primary evidence of compensatory activation patterns is provided in a study by Mürner-Lavanchy et al. (2014), who reported that increased activation in the superior prefrontal cortex might be proof of a compensatory mechanism counterbalancing underactivation in the middle frontal gyrus, typically recruited in such tasks. Thus, explaining similar performance between school-aged VPT children and controls. This study identified a bilateral fronto-parietal network subserving VSWM by late school-age, in line with previous findings (Klingberg, 2006; Thomason et al., 2009) and showed that younger underperforming preterms showed signs of decreased neural efficiency in frontal regions. However, with age performance improves substantially and the typical VSWM network is established by late early adolescence (Mürner-Lavanchy, 2014). Conversely, no frontal activation was reported in a previous fMRI study (Taylor et al., 2012) during an n-back task in a group of VPT between 7 and 9. However, the lower WM load posed of this task compared to the one used by Mürner-Lavanchy et al. (2014) might account for less frontal recruitment. Moreover, this study provided evidence of reduced activation in the PT versus the control group in the left medial parietal and right medial temporal cortices, regions typically related to both early and mature WM networks. These findings may be suggesting of a critical maturation period for working memory from 7 to 9 years. In support of this account, in a population-based study by Griffiths et al. (2013) decreased frontal activation of areas that traditionally belong to the mature VSWM network was found in 11-year-old EPT children. Lastly, signs of compensation were detected at a subcortical level in an fMRI study in late childhood that surprisingly yielded minor performance differences in a VPT sample compared to controls, but atypical activation of the caudate nucleus, which might reflect greater demand placed on VSWM (Curtis et al., 2006).
CONCLUSION
The goal of this review was to examine the recent literature on the neural correlates of visuospatial working memory in children born preterm, from infancy to adolescence. Taken together, both quantitative and qualitative changes were exhibited in the preterm WM brain netwrok. In infancy a compensatory network was employed during working memory tasks, with the preterm group showing decreased activation in core areas of VSWM circuitry as observed in typically developing children, such as the dlPFC and parieto-occipital areas (Luciana, 2003). The prefrontal cortex, in particular, is the part of the brain that undergoes prolonged maturation, which makes it vulnerable to early damage associated with preterm birth (Chafee & Goldman-Rakic, 1998). By early school-age compensatory mechanisms were already in place and between-group comparison revealed minor differences in performance (Curtis et al., 2006; Mürner-Lavanchy, 2014). Mürner-Lavanchy et al. (2014) additionally proposed that by late childhood and early adolescence the typical VSWM network is established, and despite persistent atypicalities in activation patterns probably due to the recruitment of immature neural circuits, performance was similar to that of controls, suggesting a catch-up effect at a behavioural level at least (Curtis et al., 2006). These findings are in agreement with claims that object working memory in infancy relies more on compensatory networks (e.g. hippocampus), while increasing reliance on frontal and parietal networks is established later (Bunge & Wright, 2007; Káldy & Sigala, 2004; Klingberg, 2006). Whether these deficits are transient and reflective of an immature WM network or early hippocampal lesion affects subsequent normal maturation of the core regions subserving visuospatial processing is still unknown (Nosarti & Froudist-Walsh, 2016). In adolescence, a period of increasing myelination (Scherf, Sweeney & Luna, 2006), Vollmer et al. (2017) detected no brain size differences but diffuse disruption in almost all major white matter tracts. Other diffusion tensor Imaging (DTI) studies have found that white matter is affected in brain regions that subserve intra-cortical and cortical-subcortical connections, including the corpus callosum, the superior longitudinal fasciculus and the external and internal capsules (See Li et al., 2015 for review). This abnormality in connectivity could be attributed to brain damage associated with perinatal complications of prematurity, most common being perventriculal leukomalacia(PVL). The advent of MRI has revealed that most infants with PVL exhibit a diffuse pattern of white matter tract disruption (Volpe, 2009). Moreover, some evidence suggests that long-range white matter bundles are more characteristic of the right versus the left hemisphere architecture, which is known of subserving visuospatial processing later in life (Rourke & Conway, 1997). This theory can account for the selective vulnerability observed in VSWM of preterm children (Rose et al., 2011). More evidence earlier in development comes from the findings of Clark & Woodward (2010), who proposed that neonatal white matter pathology is a stronger predictive factor of long-term VSWM deficits than grey matter abnormalities. However, such findings in childhood should be interpreted with caution since movement during scanning has been found to reduce the image of long-range connectivity in the brain, providing misleading evidence (Satterthwaite, 2012). Notably, different methodologies were employed in each study with respect to design, assessment tools, participant age and group recruitment. The tasks used tap on slightly different components of VSWM. For example, the n-back task relies exclusively on visual memory, while Corsi blocks involves a motor planning-execution component. Furthermore, the MLMS task has been argued to measure additional aspects of EF, such as inhibition, planning and cognitive flexibility (Zelazo, Reznick & Spinazzola, 1998). Additionally, not all studies controlled for general cognitive ability of their sample (e.g. Taylor et al., 2012) and two studies did not include a control group (Beauchamp et al., 2008; Scratch et al., 2015). All studies but one focused on VPT or EPT preterms, whereas Vollmer et al. (2017) had the most diverse sample, with preterms ranging from 26 to 36 weeks, which might explain why no association was found between VSWM and total white and gray matter volumes. Late preterm children have also been found to perform marginally lower or similar to their term-born peers (Baron et al., 2009). In short, imaging findings suggest different strategies between preterms and controls and recruitment of compensatory networks (Taylor et al., 2012). Thus, there is strong evidence for a deviant neurodevelopmental trajectory of this ability probably accounted by disrupted long-range connectivity due to early white matter lesions. Since WM is one of the strongest predictors of academic achievement, especially reading and mathematics (Dumontheil & Klingberg, 2011). The description of WM neurodevelopment in high-risk populations, such as those preterm, could help identify early areas of weakness and inform intervention planning so as to prevent later academic underachievement and psychosocial difficulties. Neuroimaging could potentially play a critical role in identifying children at higher risk for cognitive impairment, but to date it still unclear whether it can be a better predictor than, the undoubtedly cheaper, behavioural assessment (Gabrielli, 2009). Laslty, more large-scale longitudinal studies are needed to examine the involvement of different neural networks in VSWM throughout childhood, before the adult-level neural organization is established.
REFERENCES
Aarnoudse-Moens, C. S. H., Weisglas-Kuperus, N., van Goudoever, J. B., & Oosterlaan, J. (2009). Meta-analysis of neurobehavioral outcomes in very preterm and/or very low birth weight children.
Pediatrics
,
124
(2), 717-728. Allin, M., Henderson, M., Suckling, J., Nosarti, C., Rushe, T., Fearon, P., … & Murray, R. (2004). Effects of very low birthweight on brain structure in adulthood.
Developmental medicine and child neurology
,
46
(1), 46-53. Anderson, P. J. (2014). Neuropsychological outcomes of children born very preterm. In
Seminars in Fetal and Neonatal Medicine
(Vol. 19, No. 2, pp. 90-96). WB Saunders.Baddeley, A. (1998). Recent developments in working memory.
Current opinion in neurobiology
,
8
(2), 234-238. Anderson, P., Doyle, L. W., & Victorian Infant Collaborative Study Group. (2003). Neurobehavioral outcomes of school-age children born extremely low birth weight or very preterm in the 1990s.
jama
,
289
(24), 3264-3272. Baddeley, A. D., & Hitch, G. (1974). Working memory.
Psychology of learning and motivation
,
8
, 47-89. Barendse, E. M., Hendriks, M. P., Jansen, J. F., Backes, W. H., Hofman, P. A., Thoonen, G., … & Aldenkamp, A. P. (2013). Working memory deficits in high-functioning adolescents with autism spectrum disorders: neuropsychological and neuroimaging correlates.
Journal of neurodevelopmental disorders
,
5
(1), 14. Baron, I. S., Erickson, K., Ahronovich, M. D., Coulehan, K., Baker, R., & Litman, F. R. (2009). Visuospatial and verbal fluency relative deficits in ‘complicated’late-preterm preschool children.
Early human development
,
85
(12), 751-754. Beauchamp, M. H., Thompson, D. K., Howard, K., Doyle, L. W., Egan, G. F., Inder, T. E., & Anderson, P. J. (2008). Preterm infant hippocampal volumes correlate with later working memory deficits.
Brain
,
131
(11), 2986-2994. Bunge, S. A., & Wright, S. B. (2007). Neurodevelopmental changes in working memory and cognitive control.
Current opinion in neurobiology
,
17
(2), 243-250. Chafee, M. V., & Goldman-Rakic, P. S. (1998). Matching patterns of activity in primate prefrontal area 8a and parietal area 7ip neurons during a spatial working memorytask.
Journal of neurophysiology
,
79
(6), 2919-2940. Clark, C. A., & Woodward, L. J. (2010). Neonatal cerebral abnormalities and later verbal and visuospatial working memory abilities of children born very preterm.
Developmental neuropsychology
,
35
(6), 622-642. Curtis, W. J., Zhuang, J., Townsend, E. L., Hu, X., & Nelson, C. A. (2006). Memory in early adolescents born prematurely: a functional magnetic resonance imaging investigation.
Developmental neuropsychology
,
29
(2), 341-377. de Haan, M., Bauer, P. J., Georgieff, M. K., & Nelson, C. A. (2000). Explicit memory in low-risk infants aged 19 months born between 27 and 42 weeks of gestation.
Developmental Medicine and Child Neurology
,
42
(5), 304-312. de Kieviet, J. F., Zoetebier, L., Van Elburg, R. M., Vermeulen, R. J., & Oosterlaan, J. (2012). Brain development of very preterm and very low‐birthweight children in childhood and adolescence: a meta‐analysis.
Developmental Medicine & Child Neurology
,
54
(4), 313-323. Dumontheil, I., & Klingberg, T. (2011). Brain activity during a visuospatial working memory task predicts arithmetical performance 2 years later.
Cerebral Cortex
,
22
(5), 1078-1085. Engle, W. A. (2004). Age terminology during the perinatal period.
Pediatrics
,
114
(5), 1362-1364. Fitzpatrick, A., Carter, J., & Quigley, M. A. (2016). Association of Gestational Age With Verbal Ability and Spatial Working Memory at Age 11.
Pediatrics
, e20160578. Gabrieli, J. D. (2009). Dyslexia: a new synergy between education and cognitive neuroscience.
science
,
325
(5938), 280-283. Gathercole, S., & Alloway, T. P. (2008).
Working memory and learning: A practical guide for teachers
. Sage. Gathercole, S. E., Alloway, T. P., Willis, C., & Adams, A. M. (2006). Working memory in children with reading disabilities.
Journal of experimental child psychology
,
93
(3), 265-281. Goldenberg, R. L., Culhane, J. F., Iams, J. D., & Romero, R. (2008). Epidemiology and causes of preterm birth.
The lancet
,
371
(9606), 75-84. Griffiths, S. T., Gundersen, H., Neto, E., Elgen, I., Markestad, T., Aukland, S. M., & Hugdahl, K. (2013). fMRI: blood oxygen level-dependent activation during a working memory-selective attention task in children born extremely preterm.
Pediatric research
,
74
(2), 196-205. Gruber, O., & von Cramon, D. Y. (2003). The functional neuroanatomy of human working memory revisited: Evidence from 3-T fMRI studies using classical domain-specific interference tasks.
Neuroimage
,
19
(3), 797-809. Haxby, J. V., Petit, L., Ungerleider, L. G., & Courtney, S. M. (2000). Distinguishing the functional roles of multiple regions in distributed neural systems for visual working memory.
Neuroimage
,
11
(2), 145-156 Hamilton, C., Coates, R., & Heffernan, T. (2003). What develops in visuo-spatial working memory development?.
European Journal of Cognitive Psychology
,
15
(1), 43-69. Káldy, Z., & Sigala, N. (2004). The neural mechanisms of object working memory: what is where in the infant brain?.
Neuroscience & Biobehavioral Reviews
,
28
(2), 113-121. Klingberg, T. (2006). Development of a superior frontal–intraparietal network for visuo-spatial working memory.
Neuropsychologia
,
44
(11), 2171-2177. Lee, S. C. C. (2015).
Working Memory Performance of Children Born Preterm: The Effects of Prematurity and Training
(Doctoral dissertation, University of Alberta). Li, K., Sun, Z., Han, Y., Gao, L., Yuan, L., & Zeng, D. (2015). Fractional anisotropy alterations in individuals born preterm: a diffusion tensor imaging meta‐analysis.
Developmental Medicine & Child Neurology
,
57
(4), 328-338. Luciana, M. (2003). Cognitive development in children born preterm: implications for theories of brain plasticity following early injury.
Development and psychopathology
,
15
(4), 1017-1047. Luciana, M., Lindeke, L., Georgieff, M., Mills, M., & Nelson, C. A. (1999). Neurobehavioral evidence for working-memory deficits in school-aged children with histories of prematurity.
Developmental medicine and child neurology
,
41
(8), 521-533. Martinussen, R., Hayden, J., Hogg-Johnson, S., & Tannock, R. (2005). A meta-analysis of working memory impairments in children with attention-deficit/hyperactivity disorder.
Journal of the American Academy of Child & Adolescent Psychiatry
,
44
(4), 377-384. Mürner-Lavanchy, I., Ritter, B. C., Spencer-Smith, M. M., Perrig, W. J., Schroth, G., Steinlin, M., & Everts, R. (2014). Visuospatial working memory in very preterm and term born children—Impact of age and performance.
Developmental cognitive neuroscience
,
9
, 106-116. Nosarti, C., & Froudist‐Walsh, S. (2016). Alterations in development of hippocampal and cortical memory mechanisms following very preterm birth.
Developmental Medicine & Child Neurology
,
58
(S4), 35-45. Mulder, H., Pitchford, N. J., & Marlow, N. (2010). Processing speed and working memory underlie academic attainment in very preterm children.
Archives of Disease in Childhood-Fetal and Neonatal Edition
,
95
(4), F267-F272. Omizzolo, C., Scratch, S. E., Stargatt, R., Kidokoro, H., Thompson, D. K., Lee, K. J., … & Anderson, P. J. (2014). Neonatal brain abnormalities and memory and learning outcomes at 7 years in children born very preterm.
Memory
,
22
(6), 605-615. Rose, S. A., Feldman, J. F., & Jankowski, J. J. (2011). Modeling a cascade of effects: The role of speed and executive functioning in preterm/full‐term differences in academic achievement.
Developmental science
,
14
(5), 1161-1175. Rourke, B. P., & Conway, J. A. (1997). Disabilities of arithmetic and mathematical reasoning: Perspectives from neurology and neuropsychology.
Journal of Learning disabilities
,
30
(1), 34-46. Sansavini, A., Guarini, A., Alessandroni, R., Faldella, G., Giovanelli, G., & Salvioli, G. (2007). Are early grammatical and phonological working memory abilities affected by preterm birth?.
Journal of communication disorders
,
40
(3), 239-256. Satterthwaite, T. D., Wolf, D. H., Loughead, J., Ruparel, K., Elliott, M. A., Hakonarson, H., … & Gur, R. E. (2012). Impact of in-scanner head motion on multiple measures of functional connectivity: relevance for studies of neurodevelopment in youth.
Neuroimage
,
60
(1), 623-632. Scherf, K. S., Sweeney, J. A., & Luna, B. (2006). Brain basis of developmental change in visuospatial working memory.
Journal of cognitive neuroscience
,
18
(7), 1045-1058. Scratch, S. E., Anderson, P. J., Doyle, L. W., Thompson, D. K., Ahmadzai, Z. M., Greaves, R. F., … & Hunt, R. W. (2015). High postnatal growth hormone levels are related to cognitive deficits in a group of children born very preterm.
The Journal of Clinical Endocrinology & Metabolism
,
100
(7), 2709-2717. Shonkoff, J. P., Phillips, D. A., & National Research Council. (2000). Institute of Medicine.
From neurons to neighborhoods: The science of early childhood development. Washington, DC: National AcademyPress
. Soria-Pastor, S., Gimenez, M., Narberhaus, A., Falcon, C., Botet, F., Bargallo, N., … & Junque, C. (2008). Patterns of cerebral white matter damage and cognitive impairment in adolescents born very preterm.
International Journal of Developmental Neuroscience
,
26
(7), 647-654. Taylor, M. J., Donner, E. J., & Pang, E. W. (2012). fMRI and MEG in the study of typical and atypical cognitive development.
Neurophysiologie Clinique/Clinical Neurophysiology
,
42
(1), 19-25. Taylor, H. G., Filipek, P. A., Juranek, J., Bangert, B., Minich, N., & Hack, M. (2011). Brain volumes in adolescents with very low birth weight: effects on brain structure and associations with neuropsychological outcomes.
Developmental neuropsychology
,
36
(1), 96-117. Taylor, H. G., Minich, N., Bangert, B., Filipek, P. A., & Hack, M. (2004). Long-term neuropsychological outcomes of very low birth weight: associations with early risks for periventricular brain insults.
Journal of the International Neuropsychological Society
,
10
(7), 987-1004. Thomason, M. E., Race, E., Burrows, B., Whitfield-Gabrieli, S., Glover, G. H., & Gabrieli, J. D. (2009). Development of spatial and verbal working memory capacity in the human brain.
Journal of cognitive neuroscience
,
21
(2), 316-332. Thompson, D. K., Adamson, C., Roberts, G., Faggian, N., Wood, S. J., Warfield, S. K., … & Inder, T. E. (2013). Hippocampal shape variations at term equivalent age in very preterm infants compared with term controls: perinatal predictors and functional significance at age 7.
Neuroimage
,
70
, 278-287. Vandenbroucke, J. P., Von Elm, E., Altman, D. G., Gøtzsche, P. C., Mulrow, C. D., Pocock, S. J., … & Strobe Initiative. (2007). Strengthening the Reporting of Observational Studies in Epidemiology (STROBE): explanation and elaboration.
PLoS medicine
,
4
(10), e297. van Pareren, Y. K., Duivenvoorden, H. J., Slijper, F. S., Koot, H. M., & Hokken-Koelega, A. C. (2004). Intelligence and psychosocial functioning during long-term growth hormone therapy in children born small for gestational age.
The Journal of Clinical Endocrinology & Metabolism
,
89
(11), 5295-5302. Vollmer, B., Lundequist, A., Mårtensson, G., Nagy, Z., Lagercrantz, H., Smedler, A. C., & Forssberg, H. (2017). Correlation between white matter microstructure and executive functions suggests early developmental influence on long fibre tracts in preterm born adolescents.
PloS one
,
12
(6), e0178893. Volpe, J. J. (2009). Brain injury in premature infants: a complex amalgam of destructive and developmental disturbances.
The Lancet Neurology
,
8
(1), 110-124. Woodward, L. J., Edgin, J. O., Thompson, D., & Inder, T. E. (2005). Object working memory deficits predicted by early brain injury and development in the preterm infant.
Brain
,
128
(11), 2578-2587. World Health Organization (2016 ).
Preterm Birth
. Retrieved from http://www.who.int/mediacentre/factsheets/fs363/en/ Zelazo, P. D., Reznick, J. S., & Spinazzola, J. (1998). Representational flexibility and response control in a multistep multilocation search task.
Developmental Psychology
,
34
(2), 203.
Appendix |
||||||||||||||||||||||||||||||||||
Appendix A: Quality of studies included in the review according to the Strobe Checklist for cross-sectional, cohort and case-control studies. |
||||||||||||||||||||||||||||||||||
References |
STROBE Checklist Item No |
|||||||||||||||||||||||||||||||||
1a |
1b |
2 |
3 |
4 |
5 |
6a |
6b |
7 |
8 |
9 |
10 |
11 |
12a |
12b |
12c |
12d |
12e |
13a |
13b |
13c |
14a |
14b |
14c |
15 |
16a |
16b |
16c |
17 |
18 |
19 |
20 |
21 |
22 |
|
Woodward et al., 2005 | Y | Y | Y | Y | Y | Y | Y | C | Y | Y | Y | Y | Y | Y | Y | N | N | Y | Y | Y | N | Y | N | Y | Y | Y | Y | N | Y | Y | Y | Y | N | Y |
Beauchamp et al., 2008 | Y | Y | Y | Y | Y | Y | Y | Y | Y | Y | N | Y | Y | Y | Y | Y | N | N | Y | Y | N | Y | Y | Y | Y | Y | Y | Y | N | Y | Y | Y | Y | Y |
Clark & Woodward, 2010 | Y | Y | Y | Y | Y | Y | Y | C | Y | Y | Y | Y | Y | Y | Y | N | N | Y | Y | Y | N | Y | Y | N | Y | Y | Y | C | Y | Y | Y | Y | Y | N |
Curtis et al., 2010 | Y | Y | Y | Y | Y | Y | Y | C | Y | Y | Y | Y | Y | Y | Y | N | N | Y | Y | N | N | Y | N | Y | Y | Y | Y | N | Y | Y | Y | Y | Y | N |
Taylor et al., 2012 | Y | Y | Y | Y | Y | Y | N | C | N | Y | Y | Y | Y | Y | N | N | N | Y | Y | N | N | N | N | Y | Y | N | Y | N | Y | Y | Y | Y | Y | N |
Griffiths et al., 2013 | Y | Y | Y | Y | Y | Y | N | Y | Y | Y | Y | Y | Y | Y | Y | N | N | Y | Y | Y | N | Y | N | Y | Y | Y | Y | N | Y | Y | Y | Y | Y | Y |
Omizzolo et al., 2013 | Y | Y | Y | Y | Y | Y | Y | Y | Y | Y | N | Y | Y | Y | Y | N | Y | N | Y | Y | N | Y | N | Y | Y | Y | Y | N | Y | Y | N | Y | Y | N |
Thompson et al., 2013 | Y | Y | Y | Y | Y | N | Y | C | Y | Y | Y | Y | Y | Y | C | N | C | Y | Y | Y | N | Y | Y | C | Y | Y | Y | C | Y | Y | Y | Y | N | Y |
Mürner-Lavanchy et al., 2014 | Y | Y | Y | Y | Y | Y | Y | Y | Y | Y | Y | Y | Y | Y | Y | N | N | Y | Y | Y | N | Y | Y | Y | Y | Y | Y | N | Y | Y | Y | Y | Y | Y |
Scratch et al., 2015 | Y | Y | Y | Y | Y | Y | Y | Y | Y | Y | Y | Y | Y | Y | N | Y | N | Y | Y | Y | N | Y | N | Y | Y | Y | Y | Y | Y | Y | Y | Y | Y | Y |
Vollmer et al., 2017 | Y | Y | Y | Y | Y | Y | Y | Y | Y | Y | Y | Y | Y | Y | Y | N | Y | N | Y | Y | Y | Y | Y | Y | Y | Y | Y | N | Y | Y | N | Y | Y | Y |
Appendix B:
STROBE Statement—checklist of items that should be included in reports of observational studies
Item No | Recommendation | |
Title and abstract |
1 |
( a ) Indicate the study’s design with a commonly used term in the title or the abstract |
( b ) Provide in the abstract an informative and balanced summary of what was done and what was found |
||
Introduction | ||
Background/rationale | 2 | Explain the scientific background and rationale for the investigation being reported |
Objectives | 3 | State specific objectives, including any prespecified hypotheses |
Methods | ||
Study design | 4 | Present key elements of study design early in the paper |
Setting | 5 | Describe the setting, locations, and relevant dates, including periods of recruitment, exposure, follow-up, and data collection |
Participants | 6 |
( a ) Cohort study —Give the eligibility criteria, and the sources and methods of selection of participants. Describe methods of follow-up Case-control study —Give the eligibility criteria, and the sources and methods of case ascertainment and control selection. Give the rationale for the choice of cases and controls Cross-sectional study —Give the eligibility criteria, and the sources and methods of selection of participants |
( b ) Cohort study —For matched studies, give matching criteria and number of exposed and unexposed Case-control study —For matched studies, give matching criteria and the number of controls per case |
||
Variables | 7 | Clearly define all outcomes, exposures, predictors, potential confounders, and effect modifiers. Give diagnostic criteria, if applicable |
Data sources/ measurement | 8* |
For each variable of interest, give sources of data and details of methods of assessment (measurement). Describe comparability of assessment methods if there is more than one group |
Bias | 9 | Describe any efforts to address potential sources of bias |
Study size | 10 | Explain how the study size was arrived at |
Quantitative variables | 11 | Explain how quantitative variables were handled in the analyses. If applicable, describe which groupings were chosen and why |
Statistical methods | 12 |
( a ) Describe all statistical methods, including those used to control for confounding |
( b ) Describe any methods used to examine subgroups and interactions |
||
( c ) Explain how missing data were addressed |
||
( d ) Cohort study —If applicable, explain how loss to follow-up was addressed Case-control study —If applicable, explain how matching of cases and controls was addressed Cross-sectional study —If applicable, describe analytical methods taking account of sampling strategy |
||
( e ) Describe any sensitivity analyses |
Results | ||
Participants | 13* | (a) Report numbers of individuals at each stage of study—eg numbers potentially eligible, examined for eligibility, confirmed eligible, included in the study, completing follow-up, and analysed |
(b) Give reasons for non-participation at each stage | ||
(c) Consider use of a flow diagram | ||
Descriptive data | 14* | (a) Give characteristics of study participants (eg demographic, clinical, social) and information on exposures and potential confounders |
(b) Indicate number of participants with missing data for each variable of interest | ||
(c) Cohort study —Summarise follow-up time (eg, average and total amount) |
||
Outcome data | 15* |
Cohort study —Report numbers of outcome events or summary measures over time |
Case-control study— Report numbers in each exposure category, or summary measures of exposure |
||
Cross-sectional study— Report numbers of outcome events or summary measures |
||
Main results | 16 |
( a ) Give unadjusted estimates and, if applicable, confounder-adjusted estimates and their precision (eg, 95% confidence interval). Make clear which confounders were adjusted for and why they were included |
( b ) Report category boundaries when continuous variables were categorized |
||
( c ) If relevant, consider translating estimates of relative risk into absolute risk for a meaningful time period |
||
Other analyses | 17 | Report other analyses done—eg analyses of subgroups and interactions, and sensitivity analyses |
Discussion | ||
Key results | 18 | Summarise key results with reference to study objectives |
Limitations | 19 | Discuss limitations of the study, taking into account sources of potential bias or imprecision. Discuss both direction and magnitude of any potential bias |
Interpretation | 20 | Give a cautious overall interpretation of results considering objectives, limitations, multiplicity of analyses, results from similar studies, and other relevant evidence |
Generalisability | 21 | Discuss the generalisability (external validity) of the study results |
Other information | ||
Funding | 22 | Give the source of funding and the role of the funders for the present study and, if applicable, for the original study on which the present article is based |
*Give information separately for cases and controls in case-control studies and, if applicable, for exposed and unexposed groups in cohort and cross-sectional studies.
PLACE THIS ORDER OR A SIMILAR ORDER WITH ALL NURSING ASSIGNMENTS TODAY AND GET AN AMAZING DISCOUNT

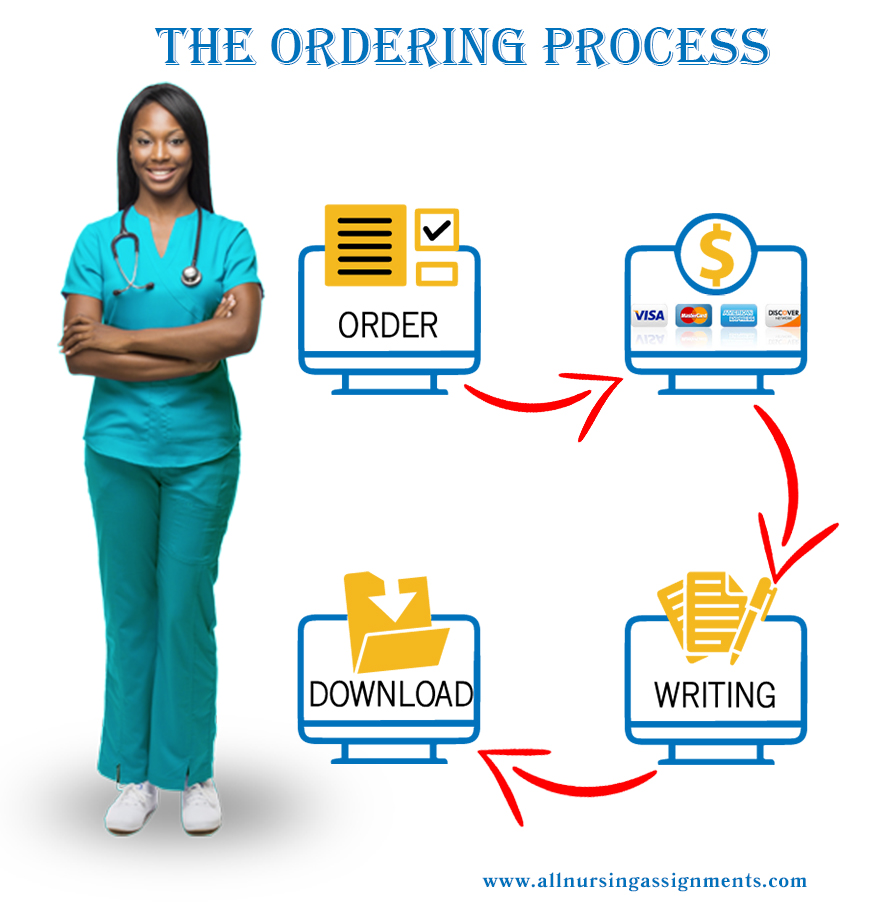