Anatomy and Physiology of Cochlear Implants
Cochlear Implants
Introduction
Hearing loss impacts a large number of people at various points in their lives
1
. Whether a parent or grandparent has a hearing aid, or a loud concert leaves the ears ringing, there is a demand for hearing improvements. According to the National Center for Health Statistics, around 18 percent of American adults reported trouble with hearing in 2012
1
. The World Health Organization estimates that 5 percent of the world’s population currently has disabling hearing loss and expects that number to grow to 10 percent by 2050
2
. People with hearing loss have used different types of hearing aids throughout history. From ear trumpets and animal horns used to funnel and amplify sound, to the electronic hearing aids of today
3
, hearing technology has come a long way. The most recent invention in hearing technology does what was once thought impossible: restore hearing in patients with sensorineural or “nerve” hearing loss
4
. Videos
5,6
have been emerging across the internet of loved ones whose hearing has been restored once a device called a cochlear implant is turned on, but the mechanism to restore their hearing is much more complicated. The implant uses electrical stimulation in different areas of the patient’s cochlea to simulate sound
4
. This paper presents a closer look at how cochlear implants work, engineering considerations in their design, and the need for biomedical engineers in their research and development.
Why Cochlear Implants are Necessary
There are two major categories of hearing loss: conductive and sensorineural
7
. Each is treated by a different device: hearing aids treat conductive hearing loss and cochlear implants treat sensorineural hearing loss. Conductive hearing loss occurs when sound waves are not able to be transmitted through the outer and middle ear due to blockages within the ear
7
. Sensorineural hearing loss occurs when the transmission of nerve impulses in the inner ear is impaired by damage to the hair cells in the cochlea
7
. The damage is usually caused by exposure to loud noises but may also be caused by various diseases, head trauma, genetics, or aging
7
. Hearing aids do not help people with sensorineural hearing loss
7
, so a different device is necessary for treatment.
History of Cochlear Implants
The development of the first cochlear implant was influenced by the discovery that electric current could convey sounds to the brain
4
. In the 1960s, three groups of scientists began to research how that discovery could be used to help patients with sensorineural hearing loss
4
. One group, William House and John Doyle, found that the strength of the stimulus affected the volume of the “sound” in the brain, and the frequency of stimulus affected the pitch
8
. These discoveries led them to create the first single channel cochlear system, meaning only one electrode was used
8
. Around the same time, the Cochlear Company built the first multichannel cochlear system, meaning an array of electrodes was used
9
. In 1982, MED-EL launched its first multichannel cochlear implant
10
. Today, the three major cochlear implant manufacturers marketed in the United States are MED-EL, the Cochlear Company, and Advanced Bionics
4
.
Anatomy and Physiology of the Ear
To understand sensorineural hearing loss and how it is rectified, the anatomy of the ear must be explained. Sound travels from the outer ear to the inner ear through a series of steps. An image of the ear’s anatomy can be found in the
Appendix
as
Figure 1
. First, sound must funnel through the pinna into the external auditory canal, and vibrate the tympanic membrane (the eardrum)
7,11
. These vibrations then pass through three bones in the middle ear: the malleus, the incus, and the stapes
7,11
. The stapes covers the oval window, which leads to the inner ear
7,11
where the cochlea is located
7,11
. The vibrations of the stapes lead to vibrations in the cochlea and the formation of action potentials that the brain interprets as sound
7
.
The cochlea is a shell-like structure that consists of a tube coiled around itself
7,11
and is composed of three channels: the scala vestibuli, the cochlear duct, and the scala tympani
7
(see
Figure 2
). The auditory nerve and the organ of Corti (the organ responsible for hearing) pass through all turns of the cochlea
7
. Vibrations pass through the scala vestibuli to the cochlear duct, causing vibrations in the tectorial membrane to move against hair cells
7
. Hair cell movement causes generator potentials when their stereocilia are displaced
7
. The potentials travel down the auditory nerve to the auditory center of the brain to be interpreted
7,11
. Different portions of the cochlea detect different frequencies, with the innermost area of the spiral detecting lower frequencies and the outermost detecting higher frequencies
7
. Depending on the frequency of the sound that travels to the ear, hair cells in the designated regions of the cochlea will be displaced
7
. The displacement sends signals to the brain that indicate a sound at a certain pitch is being heard
7
. If a sound is louder, the stereocilia bend more, which causes more frequent action potentials from that region of the cochlea
7
.
Engineering Aspects of Cochlear Implants: How They Work
Cochlear implants consist of an external microphone, processor, and transmitter, as well as an internal receiver and electrode array
7
(see
Figure 3
). The microphone takes in sound from the environment and transmits that sound to the processor
12,13
. The processor is a bit more complicated. It takes the acoustic vibrations from the microphone and converts them into electrical stimuli that the auditory nerve can interpret
12,13
. The processor sends the electrical stimulation patterns to the transmitter
14
, which is housed externally behind the ear
12-14
. The transmitter sends the signals to the receiver, which is housed internally on the temporal bone
7
, in a style known as a transcutaneous link
14
. A percutaneous connector can also be used
14
and will be discussed in the
Design Considerations
section. The receiver sends the signals to the electrode array in the cochlea, which directly stimulate different sections of the auditory nerve
12, 14
. The location of stimulus causes the electrical stimuli to be perceived as different pitches of sound
7
.
Engineering Aspects of Cochlear Implants: Design Considerations
The design considerations to be discussed include: sound coding strategies, transmission link style, electrode design and placement, and the patient. A major engineering design consideration for cochlear implants, especially in terms of improving implants
14
, is the sound coding strategy. The strategy chosen can affect speech perception, directional hearing, sound quality, and perception of music and tone
15
. There are a number of strategies to choose from, including continuous interleaved sampling (CIS), spectral peak (SPEAK), advanced combination encoder (ACE), and countless others
14
. The listed strategies are found in the three FDA-approved cochlear implant companies’ devices
14
. CIS is the simplest coding strategy used in cochlear implant design and will be explained in detail because it is the most widely used among cochlear implant manufacturers
14
. CIS was developed in response to the Compressed Analog (CA) approach, which filters and sends four different analog waveforms to four electrodes in the cochlea
16
. All of the electrodes can stimulate different parts of the cochlea at the same time, which produces problems with distortion caused by nearby electrodes
16
.
The CIS approach corrects the distortion by sending non-simultaneous, interleaved pulses to the electrodes, meaning only one electrode is stimulated at a time
16
.
Figure 4
shows a diagrammatic representation of the CIS approach. To cause the desired electrode stimulation pattern, the signal must first be pre-emphasized
16
. Pre-emphasis causes certain frequencies of the signal to be accentuated by increasing their amplitudes
17
. The pre-emphasis allows weak consonants to compete with vowels that are commonly more intense in the signal
14
. The signal is then sent through a series of band-pass filters that split it into parts
16
. Full wave rectification and low-pass filtering are used to modify the waveforms
14,16
. Full wave rectification means the signal is converted from alternating current to direct current
18
(see
Figure 5
). Low pass filtering in CIS means that frequencies of less than 200 or 400 Hz will be outputted
16
. These steps result in envelopes: smooth curves outlining the extremes of the signal
19
(see
Figure 6
). A logarithmic compression function ensures that the output envelopes will be within the range of the patient’s electrically-evoked hearing
16
. The envelopes modulate trains of biphasic pulses that are delivered to the electrodes at a non-overlapping, constant rate
14,16
. The modulation results in the biphasic pulses matching the amplitudes of the envelopes and delivered to the correct area of the cochlea
16
. The faster the biphasic pulse rate, the better the patient will recognize speech and sounds
16
.
ACE and SPEAK are both “n-of-m” strategies that split speech signals into m bands, where a subset of n bands with the largest amplitudes is then used for stimulation
20
. The goal is to only allow important signals to be heard by the patients, and implants using an n-of-m strategy have a significant user preference over CIS strategies
20
. Cochlear implant manufacturers and researchers have developed a wide number of other coding technologies that improve speech articulation, hearing in either low or high frequencies, or the ability to recognize environmental sounds
14
. A description for each of the technologies will not be given.
Another engineering design consideration for cochlear implants is whether the transmission link should be transcutaneous or percutaneous. A transcutaneous link was described in the
How They Work
section. In summary, the transmitter of the cochlear implant is external, and the receiver is internal; they are held together by magnets
14
. The components of the implant create two disadvantages for the transcutaneous link: lack of compatibility with MRI machines and the need for surgery if the implanted electronics fail
21
. In a percutaneous connection, the speech processor and stimulator are located externally, with the stimulator directly connected to both the processor and the electrodes on the cochlea
14
. The principal advantage of a percutaneous connector is that there is no need to encode the signals that are sent to the receiver
14
. However, it poses an increased risk of infection due to the opening in the skin
14
. All commercially available cochlear implants use a transcutaneous link
14
.
Electrode design and placement must also be considered when designing a cochlear implant. There are two commercially available electrode array types: straight lateral wall (LW) and pre-curved modiolar hugging (MH)
22
. Both electrode array types are inserted into the scala tympani of the cochlea
22
. The straight LW design comes in a variety of lengths, which allows for more custom fits for different cochlea sizes
22
. They stimulate nerve fiber endings of the auditory nerves at the organ of Corti, and implantation may cause damage to the cochlea
22
. The pre-curved MH design only spans to the basal turn of the cochlea and rests along the central bony wall of the inner ear
22, 23
. It stimulates the spiral ganglion cells of the auditory nerve, and implantation causes more frequent damage than straight LW electrodes
22
. However, it is easier to insert than a straight LW design
22
.
A very important design consideration is the patient
14
. Each patient has a different cochlea size and structure, with differing amounts of damage to cochlear cells
14
. For example, a pre-curved MH electrode array may not perfectly fit one person’s cochlea, so they might need a straight LW array
22
. Patients may have differing amounts of auditory nerve cells in different locations, so the cochlear implant must have electrodes that can stimulate the correct locations
14, 22
. Overall, the physician fitting the implant must be willing to identify the factors that affect the fit of a cochlear implant to the patient
14, 22
.
Engineering Aspects of Cochlear Implants: Skills for Success
Biomedical engineers are at the center of design and implementation of cochlear implants, but a large team of diverse individuals is needed for the success of the implant. The team includes physicians, psychologists, educators, entrepreneurs, engineers, and physiologists
24
. Biomedical engineers are responsible for interpreting the information from all team members in order to improve the cochlear implant
24
. The original engineers who created the implant needed to understand how the electrical components worked and how to assemble them in order to transmit sound stimulus in a way the inner ear and nervous system could interpret
4
. Therefore, the skills needed for the original design of the implant were knowledge and assembly of electrical components
4
, the anatomy and physiology of the ear, and the anatomy and physiology of the nervous system
24
. The same skills are needed in the biomedical engineers of today who are continuously improving the technology of the implant
24
. However, they also need other technical skills such as an understanding of sound coding and signal processing
14
. Biomedical engineers must work closely with electrical engineers to develop the various electrical components of the implant
4
, so they must have the ability to understand or interpret that information for others. They must also work closely with physicians to determine what needs to be improved
4
. The continuous interaction between team members with diverse backgrounds requires both oral and written communication skills in the engineer. Overall, a biomedical engineer is necessary for the design and implementation of a cochlear implant because they have both the engineering skills and medical knowledge needed to create a successful implant
24
.
Conclusion
Cochlear implants restore hearing in patients with sensorineural hearing loss
21
in a profound way. By bypassing the patient’s normal hearing mechanisms, the implant can simulate sound by directly stimulating different areas of the cochlea in the ear
21, 22
. The correct stimulation requires research
25, 26
and development to produce sound processing techniques and electrode placements that will maximize hearing restoration in patients
26
. Engineers must consider the different sound coding strategies
14, 16
, connection types
14
, electrode placements
22
, and patients
14
when designing the implant. The listed design considerations require a diverse team to interact with patients and trial different methodologies
24
. Biomedical engineers are at the center of the development process because they have the engineering skillset and the medical knowledge needed to design and construct these implants. In the future, cochlear implants may be able to completely restore the natural hearing of people with sensorineural hearing loss.
References
-
Blackwell, D. L., Lucas, J. W. & Clarke, T. C. Summary health statistics for U.S. adults: National Health Interview Survey, 2012.
National Center for Health Statistics
Vital Health Stat 10
(2014). - World Health Organization. (2018, March 15). Deafness and hearing loss. Retrieved November 8, 2018, from http://www.who.int/en/news-room/fact-sheets/detail/deafness-and-hearing-loss
-
Ealy, G. T. Of ear trumpets and a resonance plate: Early hearing aids and Beethoven’s hearing perception.
19th Century Music
17(3)
, 262-273 (1994). -
Hainarosie, M., Zainea, V. & Hainarosie, R. The evolution of cochlear implant technology and its clinical relevance.
Journal of Medicine and Life
7(Spec Iss 2)
, 1-4 (2014). - Catch the Moment. (2017, November 10). Retrieved November 08, 2018, from https://www.youtube.com/watch?v=dwCWgOpXyoo
- Bruijn, R. D. (2012, June 01). Retrieved November 08, 2018, from https://www.youtube.com/watch?v=mbe7x8GP2Ds
-
Fox, S. I. (2013).
Human physiology
(13th ed.). New York: McGraw-Hill Education. -
House, W. F. & Urban, J. Long term results of electrode implantation and electronic stimulation of the cochlea in man.
The Annals of Otology, Rhinology, and Laryngology
82(4)
, 504-517 (1973). -
Clark, G. M., Cowan, R. S. C. & Dowell, D. C. Cochlear implantation for infants and children.
Advances
(1997). -
Burian K. Letter: Significance of cochlear nerve electric stimulation in totally deaf patients.
Laryngologie, Rhinologie, Otologie
54(6)
, 530-531 (1975). -
Goelzer, B., Hansen, C. H., & Sehrndt, G. A. (2001).
Occupational exposure to noise: Evaluation, prevention and control
. Geneva: WHO. -
Rubinstein, J. T. How cochlear implants encode speech.
Current Opinion in Otolaryngology & Head and Neck Surgery
12
, 444-448 (2004). -
Dynes, S. B & Delgutte, B. Phase-locking of auditory-nerve discharges to sinusoidal
electric stimulation of the cochlea.
Hearing Research
58
, 79-90 (1992).
-
Zeng, F. G., Popper, A. N. & Fay, R. R. (2004)
Cochlear implants: Auditory prostheses and electric hearing
. New York: Springer Science+Business Media New York. -
Wouters, J., McDermott, H. J. & Francart, T. Sound coding in cochlear implants: From electric pulses to hearing.
IEEE Signal Processing Magazine
32(2)
, 67-80 (2015). -
Wilson, B., Finley, C., Lawson, D., Wolford, R., Eddington, D. & Rabinowitz, W. Better speech recognition with cochlear implants.
Nature
352
, 236-238 (1991). -
Buckwalter, J., Meghelli, M., Friedman, D. J. & Hajimiri, A. (2006). Phase and Amplitude Pre-Emphasis Techniques for Low-Power Serial Links.
IEEE Journal of Solid State Circuits
41(6)
, 1391-1399 (2006). -
Surtell, T. Analogue Electronics.
Electronics in Meccano
4
(1999). -
Caetano, M., & Rodet, X. Improved estimation of the amplitude envelope of time domain
signals using true envelope cepstral smoothing.
IEEE International Conference
on Acoustics, Speech and Signal Processing (ICASSP)
4244-4247 (2011). -
Nogueira, W., Buchner, A., Lenarz, T. & Edler, B. A psychoacoustic “NofM”-type speech coding
strategy for cochlear implants.
Journal on Applied Signal Processing
18
, 3044-3059 (2005). -
Loizou, P. C. Introduction to cochlear implants.
IEEE Signal Processing Magazine
101-130 (1998). -
Dhanasingh, A. & Jolly, C. An overview of cochlear implant electrode array designs.
Hearing Research
356
, 93-103 (2017). -
Young, N. M. & Kirk, K. I. (2016)
Pediatric cochlear implantation: Learning and the brain
. New York: Springer Science+Business Media. -
Zeng, F. G., Rebscher, S., Harrison, W., Sun, X. & Feng, H. Cochlear implants:System design, integration and evaluation.
IEEE reviews in biomedical engineering
1
, 115-142 (2008). -
Hochmair-Desoyer, I., Hochmair, E. & Burian, K. Design and fabrication of multiwire scala tympani electrodes.
Annals of New York Academy of Sciences
405
, 173-182 (1983). -
Clark, G., Shepherd, R., Patrick, J., Black, R. & Tong, Y. Design and fabrication of the banded electrode array.
Annals of New York Academy of Sciences
405
, 191-201 (1983). -
Rehman, D. Auditory Scale Analysis and Evaluation of Phonemes in MISING Language.
International Journal of Computer Applications
113(15)
1-5 (2015). -
Sorkin, D. (2016).
Cochlear Implants 2016: Advances in Technology, Candidacy and Outcomes
. -
Pikálek, T., Fořt, T. & Buchta, Z. Detection techniques in low-coherence interferometry
and their impact on overall measurement accuracy.
Applied Optics
53
8463-8470 (2014).
Appendix
Figure 1:
The anatomy of the ear
7
.
Figure 2:
A cross section of the cochlea
27
. Note: the vestibular canal and tympanic canal refer to the scala vestibuli and scala tympani, respectively.
Figure 3:
The components of a transcutaneous cochlear implant
28
.
Figure 4:
A schematic of CIS sound coding
21
.
Figure 5:
Full wave rectification when the input is a sine wave
18
.
Figure 6:
Development of a signal envelope
29
.
PLACE THIS ORDER OR A SIMILAR ORDER WITH ALL NURSING ASSIGNMENTS TODAY AND GET AN AMAZING DISCOUNT

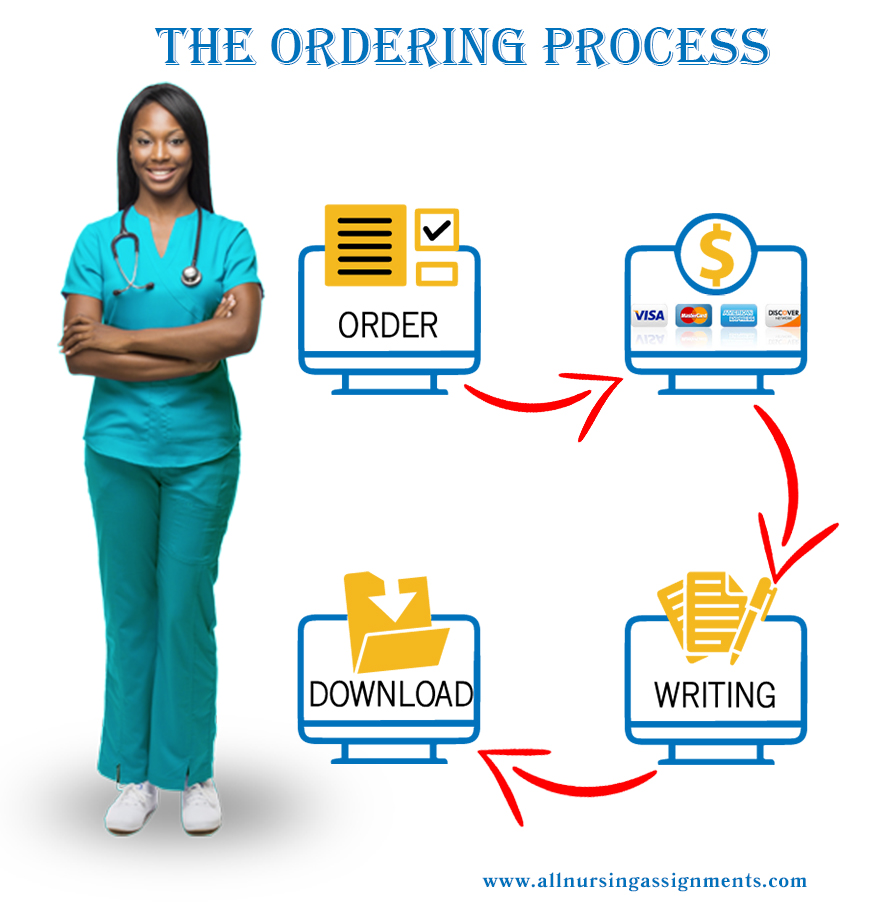